Cerebellar Nuclei and Connections in Man-Juniper Publishers
Anatomy Physiology & biochemistry International Journal (APBIJ)
The cerebellar nuclei are masses of grey matter located inside the cerebellar hemispheres. They include the fastigial, interpositus and dentate nuclei from medial to lateral. The interposed nucleus is formed of the globose nucleus medially and the emboliform nucleus laterally. The largest cerebellar nucleus is the dentate then the fastigial nucleus comes next in size. The cerebellum contains the larger number of neurons and synapses than any other part of the central nervous system. Cerebellar afferent fibres are almost three folds more numerous than its efferent fibres. They convey impulses from the periphery and different levels of the central nervous system. Most of these afferents reach the cerebellum via the inferior and middle cerebellar peduncles while less numerous afferent fibres together with efferent fibres are conveyed via the superior cerebellar peduncle. Connections and functions of the deep cerebellar nuclei as well as their structural and functional alterations in different clinical disorders are mentioned in the current article.
Keywords: Cerebellar nuclei; Morphology; Connections and functions
Abbreviations: PN: Perineuronal Net; FN: Fastigial Nucleus; DCN: Deep Cerebellar Nuclei; PCs: Purkinje Cells; PPTg: Pedunculopontine Tegmental Nucleus; DBS: Deep Brain Stimulation; RN: Red Nucleus; HRP: Horse Radish Peroxidase; PFBC: Primary Familial Brain Calcification; IBGC: Idiopathic Basal Ganglia Calcification; ODDD: Oculo Dento Digital Dysplasia; RSFC: Resting State Functional Connectivity; MS: Multiple Sclerosis; ALS: Amyotrophic Lateral Sclerosis; MRI: Magnetic Resonance Imaging; DTI: Diffusion Tensor Imaging; FRDA: Friedreich’s Ataxia; MJD: Machado-Joseph Disease; GTS: Gilles de la Tourette Syndrome; miRNAs: MicroRNAs
Introduction
The cerebellar nuclei are masses of nerve cells that are embedded inside the white matter of each cerebellar hemisphere. They are the dentate, the emboliform, the globose and the fastigial nuclei; arranged from lateral to medial.
Morphological and structural aspects of cerebellar nuclei (Figure 1)
The dentate nucleus: It is largest of all cerebellar nuclei. It is only obvious in mammals being greatly enlarged in man and apes. It is constructed of irregularly folded laminae of grey matter that are deficient antero medially to form the hilus of the nucleus. This hilus allows the emergence of the white matter fibres from inside the nucleus. These fibres represent the myelinated axons of the nerve cells of the nucleus and are continued upward to form the main bulk of the superior cerebellar peduncle. The shape of the dentate nucleus, in cross section, simulates that of the inferior olivary nucleus of the medulla oblongata. Its neurons are mainly large multipolar cells with branching dendrites. Among these large cells small stellate cells are also found in the nucleus. The mean values of length and breadth of the dentate nucleus were estimated, in Bangladeshi cadavers of both sexes, to be 8.619±2.995mm and 14.770±3.604mm respectively and these values increased with the advance of age then became slightly decreased in late ages. Significant differences of each of the mean length and breadth of the dentate nucleus on both sides were observed between different age groups while statistically insignificant differences between male and female existed [1]. Rhombencephalosynapsis is a hindbrain malformation featured by complete or partial absence of the cerebellar vermis with variable degrees of midline continuity of the cerebellar hemispheres, dentate nuclei, and sometimes the superior cerebellar peduncles. Prenatal diagnosis of this condition is reported to be feasible by ultrasound and magnetic resonance imaging [2].
The emboliform nucleus: It is formed of clusters of cells resembling thos of the dentate nucleus. It is located close to the medial side of the dentate nucleus overlapping a part of its hilus.
The globose nucleus: It lies between the fastigial nucleus medially and the emboliform nucleus laterally. It is formed of more than one rounded grey matter masses; this is why sometimes termed as the “globose nuclei”. Despite their name, they are elongated anteroposteriorly. In lower mammals, the emboliform and globose nuclei form a single grey matter mass termed the “nucleus interpositus or interposed nucleus”. There are controversies whether learning of conditioned eye blink responses primarily takes place within the cerebellar cortex, the interposed nuclei, or both. It has been suggested that the cerebellar cortex may be important during early stages of learning, and that there is a shift toward the cerebellar nuclei during later stages. Event-related MRI signals are observed to be increased concomitantly in the cerebellar cortex and nuclei during early acquisition of conditioned eye blink responses [3].
The fastigial nucleus: It is larger than each of the emboliform and globose nuclei but smaller than the dentate nucleus. It is located close to the midline, within the anterior part of the vermis in the roof of the fourth ventricle. It is composed of densely clumped nerve cells of variable sizes. The large, medium and small cells are intermingled together in the dorsal pat of the nucleus while the ventral part of the nucleus is mainly formed of small-sized cells. Afferent fibres, arising from the Purkinje cells of the cerebellar cortex, are projected to the deep cerebellar nuclei. Fibres from the anterior and posterior parts of the cerebellar vermal cortex are conveyed to both fastigial nuclei while fibres from the paravermal cortex are mostly projected to the ipsilateral globose and emboliform nuclei. Few fibres from the paravermal cortex go to some parts of the dentate nucleus. Fibres from the lateral cerebellar cortex pass to the whole length of the dentate nucleus, to the caudal parts of the nucleus interpositus, and to both fastigial nuclei. A net-like extracellular matrix around distinct neurons throughout the whole CNS is called the perineuronal net (PN). PN surrounds the cell body, proximal dendrites, and the axonal initial segment embedding synaptic terminals on the neuronal surface. These PNs are suggested to support the synaptic stabilization, to inhibit the axonal sprouting, and to control the neuronal plasticity. In a mouse model for Purkinje cell degeneration, the effect of deafferentation on synaptic remodeling and modulation of PNs in the deep cerebellar nuclei had been studied by some investigators [4]. Some authors stated that glutamergic neurons in the cerebellar fastigial nucleus (FN) regulate innate and adaptive immune functions and the immuno modulation is conveyed by FN-hypothalamic glutamatergic projections as well as sympathetic nerves that innervate lymphoid tissues in the spleen and mesenteric lymphoid nodes [5]. Death and production of the deep cerebellar nuclei (DCN) neurons were studied in the weaver condition of mutant mice. The deficit of DCN neurons was always more pronounced in the homozygous weaver mice than in the heterozygous ones. In the mediolateral axis, neurodegeneration was more profound in the medial parts than in the lateral ones. No loss of neurons was found in the dentate nucleus. In the rostrocaudal axis, neuronal loss in the fastigial nucleus exceeded that in the interposed nucleus which, in turn, exceeded the loss in the dentate nucleus. In a given deep cerebellar nucleus, the rostral region always presented more late-born neurons than the middle region and this, in turn, was later than the caudal region [6].
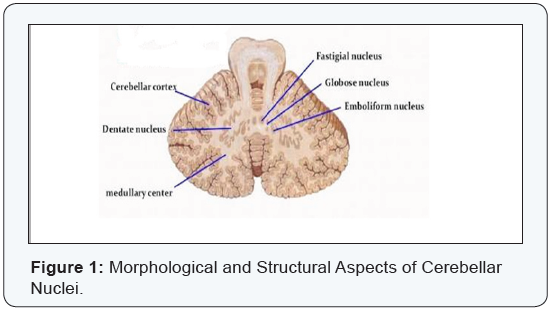
Functional and clinical aspects of cerebellar nuclei
The cerebellum contains the largest number of neurons and synapses of any structure in the central nervous system. The neurons of the cerebellar nuclei are influenced by a set of inputs. Excitatory inputs are exerted by many mossy fibres derived from the pontine nuclei and climbing fibres originating from the inferior olivary nucleus. Vast inhibitory inputs arise from the Purkinje cells located in the cerebellar cortex. Integration between these excitatory and inhibitory inputs is essential in order to generate the sole output signals of the cerebellum. The strong inhibitory input of the cerebellar nuclei is counteracted by depolarizing potentials in the recipient cerebellar nuclei cells that seem to show spontaneous depolarization activity most of the time even in the presence of such a strong inhibition. This spontaneous depolarization derive may be supplied by the excitatory mossy and climbing fibres. However, such an activity is not abolished on cutting these excitatory fibres in vitro; this has led to the assumption that the depolarizing tendencies of the cerebellar nuclei cells are attributed to intrinsic mechanisms rather than to external excitatory input. The majority of synapses onto the deep cerebellar nuclei (DCN) neurons are reported to be derived from Purkinje cells (PCs), suggesting that PC activity is an essential determinant of DCN firing [7].
Cerebellar Connections
Afferent fibres
Afferent cerebellar fibres are almost three folds more numerous than the efferent fibres. They convey impulses from the periphery and different levels of the central nervous system through some relay nuclei in the brainstem. Most of these afferent fibres reach the cerebellum via the inferior and middle cerebellar peduncles while less numerous fibres are conveyed through the superior cerebellar peduncle [8]. The nature and function of the pedunculopontine tegmental nucleus (PPTg) projections to cerebellar nuclei is still unclear. These fibers may play an essential role in postural control and may be involved in the beneficial effects induced by deep-brain stimulation (DBS) of brainstem structures in motor disorders. Some investigators surveyed the microstimulation of the PPTg on the dentate, fastigial and interpositus nuclei in rats. The dentate nucleus was the most responsive to PPTg stimulation. Destruction of the PPTg cells had reduced the PPTg-evoked activation of the dentate neurons. Application of anticholinergic agents also reduced or eliminated the PPTg-evoked response recorded in the dentate nucleus [9].
The inferior cerebellar peduncle is formed of two portions: a larger lateral entirely afferent portion named the “restiform body” and a smaller medial portion, containing both afferent and efferent fibres, named the “juxtarestiform body”. The latter conveys the vestibulecerebellar and cerebello vestibular pathways. All afferent fibres go to the cerebellar cortex except the vestibulocerebellar, olivocerebella and some rubrocerebellar that go to the deep cerebellar nuclei. The afferent fibres, directly or indirectly connecting the periphery to the cerebellum, convey impulses from the vestibular apparatus as well as proprioceptive impulses from stretch receptors in the skeletal muscles and tendons. In the cerebellar vermis, the leg is represented in the central lobule, the arm in the culmen and the head more caudally. In the paravermal area, the head area is the most rostral while the leg one is the most caudal. Auditory and visual impulses are conveyed to limited areas in the folium, tuber and close portions of the cerebellar hemisphers.
Direct and indirect vestibulocerebellar tracts: They play an important role in maintenance of equilibrium and coordination of eye movements. They originate from the labyrinth. Direct vestibulocerebellar begins at the vestibular ganglion of the vestibular portion of the vestibulocochlear nerve and ends at the ipsilateral part of the flocculonodular lobe (vestibular or archicerebellum). The indirect vestibulocerebellar tract relays in the vestibular nuclei in the brainstem before reaching the cerebellar flocculus. In a study of dorsal medullary infarction, all patients had shown acute isolated vestibular syndrome with dizziness/vertigo and imbalance. In addition, almost all patients revealed signs resulting from involvement of the vestibular nuclei, nucleus prepositus hypoglossi, or inferior cerebellar peduncle in the form of different types of nystagmus [10]. Isolated vestibular syndrome may occur along the vestibular pathways from the peripheral labyrinth to the brain. Apart from the five distinct types of isolated central vestibular syndrome resulting from lesions restricted to the vestibular nuclei, the nucleus prepositus hypoglossi, the flocculus, the tonsil, and the nodulus, a new vestibular syndrome from isolated involvement of the inferior cerebellar peduncle has been described. The latter may present with decreased responses to head impulses and possibly falsely negative MRI during the acute phase of isolated vestibular syndrome caused by a stroke [11].
Dorsal (direct) spinocerebellar tract: It conveys pro prioception and fine touch, through the inferior cerebellar peduncle, to the paleocerebellum (spinal cerebellum) in the ipsilateral part of the anterior lobe, the pyramis and the uvula [12].
Ventral (indirect) spinocerebellar tract: It ascends through the spinal cord and the brainstem to enter the superior cerebellar peduncle; being located dorsolaterally then they wind medially around the main efferent bulk of the peduncle, to end mainly in the contralateral paleocerebellum [13].
Rostral spinocerebellar tract: It arises from cells rostral to the dorsal nucleus of Clarke in the spinal cord and ascends in the anterior part of the spinal cord. Its fibres reach the cerebellum via the superior and inferior cerebellar peduncles to be distributed to its anterior lobe [14].
Olivocerebellar tract: It forms the main mass of the inferior cerebellar peduncle. It arises from the inferior olivary nucleus in medulla oblongata. Its fibres are distributed to all parts of the contralateral cerebellar cortex. Fibres from the medial part of the inferior olivary nucleus and from the accessory olivary nucleus pass to all portions of the vermis. The olivary system is highly developed in man. The inferior olivary nucleus gives rise to climbing fibres that have strong excitatory synapses on the dendrites of the cerebellar Purkinje cells. The main part of the inferior olivary nucleus receives fibres from the descending tegmental tract; these fibres arise from the red nucleus, the central grey matter and the tegmentum of the midbrain. In addition, spinoolivary fibres ascend to the medial and dorsal accessory olivary nuclei and they have been physiologically recognized by their climbing fibre responses in the cerebellar anterior lobe. In rodents, the olivo-cerebellar system has been suggested to play an essential role in the detection and adaptive correction of movement errors [15].
Ventral and dorsal external arcuate fibres: They are wandering fibres going to the cerebellum. The dorsal fibres (cuneocerebellar tract) arise from the accessory cuneate nucleus that lies dorsal to the cuneate nucleus in the lower medulla. The ventral fibres arise from the arcuate nucleus that lies ventral to the pyramid. These arcuate fibres are conveyed, via the inferior cerebellar peduncle, to the posterior part of the anterior lobe, the paramedian lobule, and the bottom of the prepyramidal fissure in the posterior part of the vermis.
Reticulocerebellar fibres: They arise from the lateral and paramedian reticular nuclei in the medulla oblongata. They reach the cerebellum via the inferior cerebellar peduncle. The lateral reticular nucleus projects fibres to the ipsilateral cerebellar anterior lobe and paramedian lobule. Moreover, this lateral nucleus receives descending fibres from the red nucleus in the midbrain and feedback cerebelloreticular fibres from the fastigial nucleus. The paramedian reticular nuclei project fibres which are mostly uncrossed to the vermis of the anterior lobe, the pyramis, and the uvula; some fibres play a role in the cerebellar feedback. The reticulotegmental nucleus of the pons receives a bilateral projection from the frontal cortex and an ipsilateral projection from the parietal cortex as well as a part of the cerebellar output via the descending part of the superior cerebellar peduncle. This nucleus seems to be a part of the reticular feedback system. Cerebellar control of movements is found to be dependent on mossy fiber input conveying information about sensory and premotor activity in the spinal cord. The lateral reticular nucleus provides the major mossy fibre input to cerebellum, from spinal inter neuronal systems to the deep cerebellar nuclei, exerting a direct excitatory effect on descending motor pathways and playing animportant role in cerebellar motor control [16,17].
Coticopontocerebellar fibres: The cortico pontine fibres arise from the cortex of the frontal and temporal lobes of the cerebral hemisphere and to a lesser extent of the parietal and occipital lobes, to reach the pontine nuclei. Fibres from the frontal cortex terminate into the cranial pons while those from the temporal cotex go to the caudal pons. The pontocrebellar fibres are mostly crossed and together with the uncrossed fibres form the main bulk of the middle cerebellar peduncle going to the cerebral cerebellum (neo cerebellum), the paramedian lobe, the folium, the tuber and all other parts of the vermis except the nodulus. This means that although the main mass of the pontocerebellar fibres go to the neocerebellum, a considerable number of fibres are projected to the paleocerebellm [18].
Trigeminocerebellar fibres:
They arise from the three sensory nuclei of the trigeminal nerve. The primary trigeminocerebellar fibres arise from the mesencephalic nucleus and pass through the superior cerebellar peduncle to be distributed to the dentate and emboliform nuclei; thus conducting proprioceptive impulses from the stretch receptors in the muscles of mastication (and possibly from the facial muscles). The secondary trigeminocerebellar fibres arise from the main sensory (principal) nucleus and the spinal nucleus of the trigeminal nerve, pass through the inferior cerebellar peduncle and terminate in the culmen and declive parts of the superior vermis [18].
Tectocerebellar fibres: They arise from the superior and inferior corpora quadrigemina (colliculi). They pass through the superior cerebellar peduncle to reach the cerebellar cortex. They carry impulses related to visual and auditory responses.
Efferent fibres
The cerebellar efferent fibres are organized into two major systems: the superior cerebellar peduncle the fastigial efferent projection. The superior cerebellar peduncle (brachium conjunctivum) is the largest cerebellar efferent bundle. It contains fibres that arise from the dentate, emboliform and globose nuclei. Fibres from the dentate nucleus emerge from its hilus, pass cranially, decussate at the level of the inferior colliculus of the midbrain and terminate into the upper part of the pons. Most of these crossed fibres end by surrounding the contralateral red nucleus in the midbrain (dentatorubral fibres) and some fibres project to the the ventral lateral nucleus of the thalamus (dentatorubrothalamic fibres). Impulses from the dentate nucleus are mentioned to influence the activity of the motor cerebral cortex that sends impulses, via the corticospinal tract, to different segments of the spinal cord; thus coordinating somatic motor function. Fibres from the emboliform and globose nuclei also pass through the superior cerebellar peduncle, decussate to terminate around the caudal portion of the conralateral red nucleus. Some investigators hypothesized that activation of cerebellar cortex and dentate nucleus had increased with increasing central executive working and memory demands [19,20]. Target recognition by developing axons is one of the fundamental steps for establishment of the proper pattern of neuronal connectivity during development. In a study investigating how commissural axons in mice recognize their targets after crossing the midline, deep cerebellar nuclei (DCN) axons, when projected to the red nucleus (RN) during development, initially enter the RN at its caudal end and continue rostrally through the RN without showing signs of axon branching. Interestingly, after some delay, DCN axons start forming interstitial branches from the portion of the axon shaft within the RN which is considered one of the well-characterized targets of DCN axons. It has been concluded that mechanism of RN recognition by DCN axons involves a delayed interstitial branching, and that these axons possess an intrinsic ability to respond to the target-derived cues irrespective of midline crossing [21]. The posterior hypothalamic nucleus is described as an area controlling autonomic activity. Using the horseradish peroxidase (HRP) retrograde axonal transport technique in rat, the afferent input to the posterior hypothalamic nucleus from cortical, sub cortical and brainstem structures has been traced. This nucleus is found to receive projections from the lateral and medial cerebellar nuclei and from upper cervical spinal levels, allowing an understanding of how this nucleus controls visceral function [22]. It is reported that there are structural similarities between the cerebellar cortex and the dorsal cochlear nucleus (the 1st station in the mammalian auditory pathway). There are cerebellar-like natures of the superficial layers of the dorsal cochlear nucleus and uni polar brush cells that are unique to certain regions of the cerebellar cortex and to the granule cell layers of the cochlear nuclei [23]. The cochlear nucleus projection to the pontine nuclei has been considered as the necessary auditory pathway for cerebellar learning including eye blink conditioning [24]. Symmetrical (bilateral) calcification of the cerebellar nuclei, basal ganglia, and other deep cortical structures have been detected in primary familial brain calcification (PFBC), also referred to as Idiopathic Basal Ganglia Calcification (IBGC) or “Fahr’s disease”. The clinical features are attributed to dysfunction of the calcified anatomical localities [25]. Oculodentodigital dysplasia (ODDD) is a rare disorder that is featured by syndactyly, microphtalmia, microdontia, and neurological disturbances rarely associated with massive calcifications of the basal ganglia and cerebellar nuclei. This gross calcification has been explained on the basis of altered brain micro vessels that lead to massive calcifications, as in the primary familial brain calcification [26]. Modifications of cerebellar resting state functional connectivity (RSFC) of the dentate nucleus have been observed in paediatric multiple sclerosis (MS) and they are influenced by the duration of the disease and brain focal lesions. Decreased RSFC may reflect early maladaptive plasticity contributing to cognitive impairment [27]. Neurodegenerative process in amyotrophic lateral sclerosis (ALS) has been observed to involve several cortical and sub cortical brain regions within and beyond motor areas. This neuro degeneration propagates along the axonal pathways to progressively involve the fronto temporal regions with their afferents and efferents, and to induce grey matter atrophy (including that of the cerebellar hemisphers) in earlier stages then it disappears in later stages probably as a result of reactive gliosis [28]. In Old World monkeys, inputs to primary motor cortex (M1) from thalamic divisions connected with cerebellum, were observed to constitute three fold the density of inputs from divisions connected with basal ganglia, with a conclusion that cerebellar nuclei are likely more involved than basal ganglia nuclei with the contributions of rostral M1 to manual dexterity [29]. Spasticity represents a common and very often incapacitating neurologic condition. Stereotactic ablation of the dentate cerebellar nuclei (dentatotomy) has been widely used as a relatively safe procedure that should still be adopted in specific cases of spasticity [30]. The fastigial efferent projections emerge from the cerebellum through the fasciculus uncinatum and the juxta restiform body. In the fasciculus uncinatum, fibres originating from the rostral part of the fastigial nucleus are uncrossed and they largely project to the vestibular nuclei while the more numerous fibres from the caudal part of the same nucleus are mostly crossed. In the descending part of the uncinate fasciculus, the fibres pass ventromedially to end into the vestibular nuclei and the reticular formation of the pons and medulla. Some fibres in this bundle bypass the vestibular nuclei to reach the reticular formation exerting inhibitory functions. Direct cerebellovestibular fibres, originating from the Purkinje cells of the vermis of the cerebellar anterior lobe, are projected to the lateral and inferior vestibular nuclei and they also exert inhibitory functions. Neurons in the cerebellar cortex, cerebellar nuclei, and medullary inferior olive form a trisynaptic loop critical for motor learning. Inferior olivary neurons excite cerebellar Purkinje cells via climbing fibres and depress their parallel fibre inputs. Purkinje cells inhibit diverse cells in the cerebellar nuclei [31]. Some descending fibres could be traced to the lateral reticular nucleus of the medulla and to the perihypoglossal nuclei. In the small ascending part of the uncinate bundle some fibres, arising from the caudal part of the fastigial nucleus and decussating inside the cerebellum, pass in the dorsolateral part of the brainstem to reach the contralateral thalamic nuclei particularly the ventral lateral nuleus. It is hypothesized that outgoing motor commands, computed by the cerebellum, use corollary discharge or copies to accelerate motor corrections. The pathway from the cerebellar nuclei to the cerebellar cortex, in mice, has been noticed to include collaterals of cerebellar pre motor output neurons [32]. Studies have shown that fastigial nucleus stimulation (FNS) reduces tissue damage resulting from focal cerebral ischemia. MicroRNAs (miRNAs) are a newly discovered group of non-coding small RNA molecules that are involved in the regulation of cell proliferation and cell apoptosis. A novel miRNA, referred to as rno-miR-676-1, was identified in the rat ischemic cerebral cortex after one hour of FNS with the suggestion that this novel miRNA may have antiapoptotic effects and it contributes to neuro protection induced by FNS [33]. Other studies have demonstrated that ingestion of the emetic compound copper sulfate (CuSO4) alters the responses to vestibular stimulation of a large fraction of neurons in brainstem regions that mediate nausea and vomiting, therefore affecting motion sickness susceptibility. The processing of vestibular inputs by cerebellar neurons plays a critical role in generating motion sickness. The neurons in the cerebellar fastigial nucleus are mentioned to receive visceral inputs. Convergence of gastrointestinal and vestibular inputs occurs mainly in the brain stem [34]. Vestibular-evoked myogenic potentials (VEMPs) are short latency manifestations of vestibuloocular and vestibulocollic reflexes that originate from the utricle and saccule. Patients with unilateral cerebellar infarctions may show abnormal VEMPs especially when they have the ocular tilt reaction [35]. The influence of age and sex on iron levels in deep grey matter magnetic susceptibilities has been investigated in healthy volunteers. Brain iron accumulated in the caudate nucleus, putamen, globus pallidus, thalamus, pulvinar, red nucleus, substantia nigra and cerebellar dentate nuclei, with the result of diverse age and sex related changes [36]. Structural and functional magnetic resonance imaging (MRI) and diffusion tensor imaging (DTI) have revealed that the thalamus, with its cortical, subcortical, and cerebellar connections, has showed a decline in cognitive functions with normal aging. This includes component processes of memory and executive functions of attention and information processing [37].
Spinal cord and peripheral nerves are well known to be damaged in patients with the most common hereditary ataxia; Friedreich’s ataxia(FRDA). These patients present with more widespread grey and white matter damage, including infra tentorial and supratentorial structures [38]. In FRDA, thinning out of the cervical spinal cord is a consistent observation in magnetic resonance imaging (MRI) and neuro pathological examination reveals neuronal loss in grey matter nuclei and degeneration of white matter tracts in the spinal cord, brainstem and cerebellum. The greatest degeneration has been observed in the left superior cerebellar peduncle, left posterior thalamic radiation, major forceps, left inferior fronto-occipital associative fasciculus and corpus callosum [39]. The spinocerebellar ataxias types 2 (SCA2) and 3 (SCA3) are autosomal dominantly inherited cerebellar ataxias. Postmortem studies have revealed a consistent neuro degeneration of the dopaminergic substantia nigra in patients with SCA2 and with SCA3. However these patients do not clinically exhibit parkinsonism except rarely. Consistently, an affection of the thalamic ventral anterior and ventral lateral nuclei, the pallidum and the cholinergic pedunculopontine nucleus has been always observed [40]. In spinocerebellar ataxia type 3, also known as Machado-Joseph disease (MJD), there are deficits of gaze holding and ocular alignment. Strabismus, intermittent exotropia, esotropia, skew deviation, hypotropia, and exophoria are among the manifestations of this disease. It seems that involvement of the brainstem, the deep cerebellar nuclei, and the superior cerebellar peduncle are the underlying pathophysiological basis for such manifestations [41].
Considering the neurophysiology of the cerebellar system, some authors have classified degenerative ataxias into four types depending on which system is affected: Purkinje cells, corticopontocerebellar, spinocerebellar and cerebellar deep nuclei systems. In order to regulate voluntary movements, the cerebellum forms internal models within its neural circuits that mimic the behaviour of the sensorimotor system and objects in the external environment. It is speculated that loss of Purkinje cells leads to malformation of the internal cerebellar models, whereas disturbance of the afferent system, corticopontocerebellar system or spinocerebellar system results into mis-selection of the proper internal model [42,43].
Spinocerebellar ataxias types 3 and 6, and Friedreich’s ataxia are common hereditary ataxias with a consistent finding of atrophy of the cerebellar cortex. The volume of the cerebellum is markedly reduced in spinocerebellar ataxia type 6, mildly reduced in spinocerebellar ataxia type 3 and preserved in Friedreich’s ataxia. The volume of the cerebellar nuclei is reduced in all the three types of ataxias, being most profound in spinocerebellar ataxia type 6 [44]. Experimentally, the neurotoxin 3-acetylpyridine (3-AP) was intraperitoneally injected in rats to induce cerebellar ataxia. All the three cerebellar nuclei (fastigial, interposed and dentate) of the cerebellar ataxia rats had shown a neuronal loss and a decrease in the neurotransmitter content. Interferon- and interleukin-producing cells were also decreased. On the contrary, B lymphocyte number, and IgM and IgG antibody levels were elevated. A conclusion that cerebellar ataxia alters cellular and humoral immunity has been assumed [45]. In ethanol consumption, the cerebellum proves to be an important target organ and the cerebellar ataxia is the most consistent physical manifestation of acute ethanol intoxication [46]. In another study on rats, curcumin which is the main ingredient of the turmeric has been mentioned to prevent the structural changes induced in the deep cerebellar nuclei by sodium metabisulfite, a preservative agent [47]. Furthermore, an experimental study on rats has shown that α-tocopherol alone and the combination of allicin and vitamin B-complex, used as adjuvant therapies, could exert a protective role against lead-acetate neurotoxicity on the cerebellar cortex [48].
Metronidazole is widely used in treatment of anaerobic bacterial and protozoal infections. Two Japanese cases of metronidazole-induced encephalopathy were detected during treatment of brain abscess. MRI showed hyperintensities within the bilateral dentate nuclei and corpus callosum. On metronidazole withdrawal, symptoms and abnormal MRI signals had completely recovered [49]. Wilson’s disease is an inborn error of metabolism characterized by excessive deposition of copper into the eyes, liver and brain tissues. Lentiform nuclei are the most involved structures, but atrophy of cerebral and cerebellar cortices is also evident [50]. Clinical and experimental evidence for epilepsy suggests a role of the cerebellum in seizure control. An epileptogenic state in rats has been characterized by a decreased activity of the deep cerebellar nuclei, particularly the dentate and fastigial nuclei. Restoration of fastigial nucleus activity, by low frequency electrical stimulation, is suggested as a possible option for treatment of chronic epilepsy [51]. Generalized epilepsy is characterized by recurrent seizures caused by oscillatory neuronal firing throughout thalamocortical networks. Therapeutic options include intervention at the level of the thalamus or cerebral cortex to ameliorate seizures. Stimulation of the deep cerebellar nuclei has been observed to effectively stop the generalized seizures in experimental models [52]. In patients with essential tremor, dentate nucleus activation proportionally increases with tremor severity. These patients also have reduced activation in widespread cerebellar cortical regions, the inferior olivary nucleus, and the parietal and frontal cerebral cortex [53]. Similar studies have been tried on animal models [54]. Gilles de la Tourette syndrome (GTS) is a neuropsychiatric disorder in which certain circuits in the cerebellum may undergo learning-induced changes. The cells of the cerebellar cortex appear to decrease their activity while those of the deep cerebellar nuclei seem to do the reverse [55]. The cerebellum is not only involved in fine motor function but also in higher cognitive functions including language. In autism, cerebellar deficits are implicated as the computational power of the cerebellum is needed for most of the processes that are disturbed in autism including language and communication, social interactions, stereotyped behavior, motor activity and motor coordination, and higher cognitive functions [56].
For more Open Access Journals in Juniper Publishers please
click on: https://juniperpublishers.com
For more articles in Anatomy Physiology & Biochemistry International Journal please click on: https://juniperpublishers.com/apbij/index.php
For more Open Access Journals please click on: https://juniperpublishers.com
To know more about Juniper Publishers please click on: https://juniperpublishers.business.site/
For more articles in Anatomy Physiology & Biochemistry International Journal please click on: https://juniperpublishers.com/apbij/index.php
For more Open Access Journals please click on: https://juniperpublishers.com
To know more about Juniper Publishers please click on: https://juniperpublishers.business.site/
Comments
Post a Comment